Proteolysis targeting chimeras (PROTACs or degraders) are a promising therapeutic approach currently undergoing clinical trials. They work by selectively breaking down unwanted proteins through ternary complex (TC) formation, driving proteasomal degradation. Understanding the 3D structure of these complexes is vital for PROTAC drug design. Enter DegraderTCM—a novel computational method that efficiently models PROTAC-mediated TCs with high accuracy using minimal computational resources. Validated against experimental structures, DegraderTCM predicts PROTAC degradation activity based on the TC structure, enabling modeling of TCs for significant degraders and guiding the design of new PROTAC drugs. Analyzing structure-activity relationships unveils potential for early-stage utilization of DegraderTCM in novel PROTAC design.
Figure one
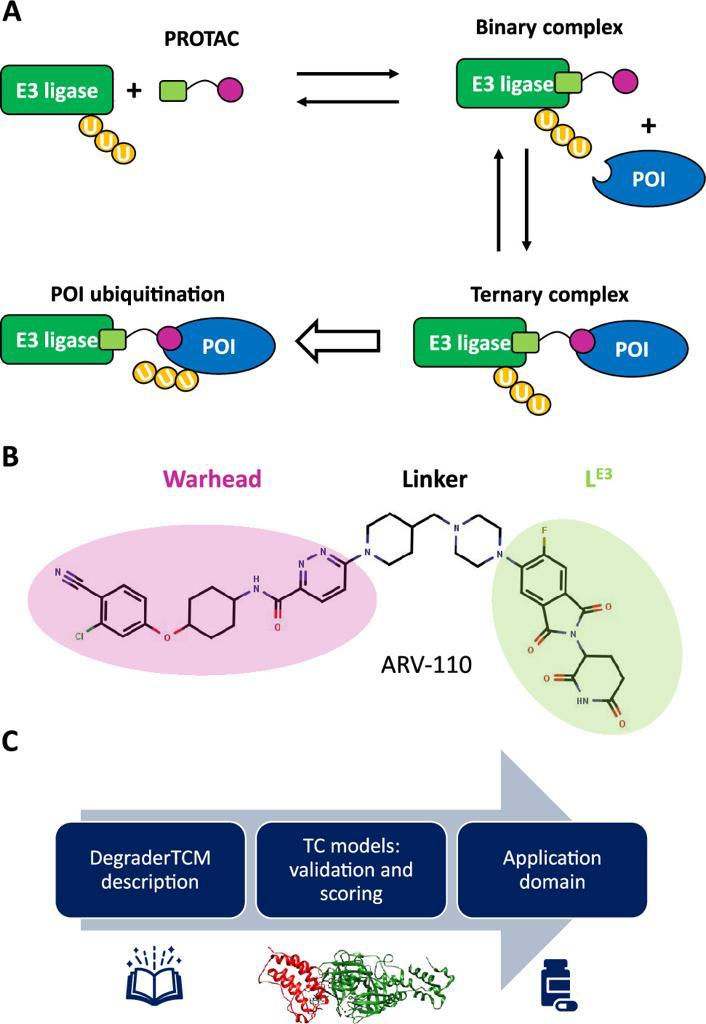
PROTACs offer a versatile approach for degrading unwanted proteins, enabling interventions against oncogene overexpression or treating neurodegenerative disorders by eliminating misfolded proteins. Notably, after fulfilling its role, each PROTAC molecule can engage with other E3 and POI units, displaying a catalytic effect. This property, where a single PROTAC molecule triggers degradation of multiple POI units, demonstrates substoichiometric behavior, enabling lower doses during administration and reducing off-target effects. Moreover, the lower warhead affinity is adequate for promoting degradation even in the absence of active sites, allowing the potential targeting of traditionally challenging proteins, such as transcription factors and scaffolding proteins.
Figure two
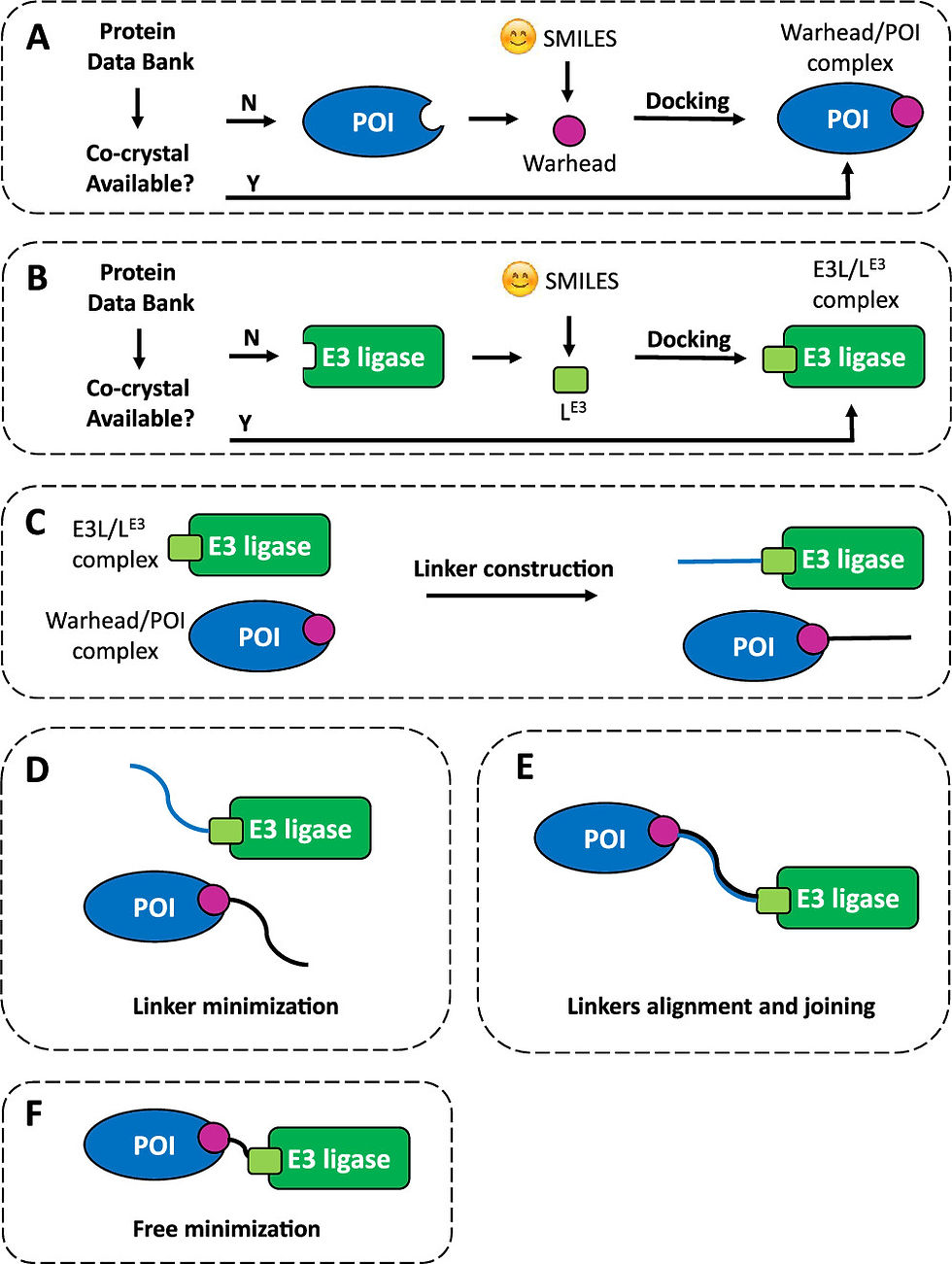
The process began by considering the POI–warhead complex (Figure 2A). When available, a co-crystal structure from the PDB was used; otherwise, the warhead pose was obtained via a docking procedure in MOE (refer to SI for details). Similarly, the procedure was applied to the E3L–LE3 complex in the second step (Figure 2B).
Next, the linker was constructed de novo at its full length using the MOE builder function and separately attached to both the warhead and E3L–LE3 while maintaining the identified binding poses (Figure 2C). These complexes underwent separate minimization (Figure 2D) to orient the linkers, reduce clashes, and position them solvent-facing—a crucial step for subsequent processes.
The two minimized complexes were merged to form the TC (Figure 2E) by superposing the linkers with MOE's superpose tool, removing excess atoms, and connecting the PROTAC structure, thus obtaining an approximate TC model.
In the final step (Figure 2F), a series of minimization cycles were performed for refinement. Protein clashes were identified and resolved through local minimization rounds. Subsequently, the PROTAC molecule was minimized to fit within the proteins. Finally, unrestrained minimization of all system atoms generated the ultimate TC model. The MOE structure preparation tool verified the absence of residual clashes.
The last minimization phase often led to reciprocal movement of the protein structures, forming new contacts. A preliminary visual inspection of the PROTAC was informative, aiding in verifying optimal binding poses for the warhead and LE3.
For validation, five TCs with available crystallographic structures in the PDB were chosen. Table 1 presents the PDB codes, resolutions, PROTAC structures, and POI/E3 pairs of these TCs. These selections were based on their use in validating other methods, their representation of PROTAC chemical diversity (particularly in linkers), and the involvement of different E3 ligases. Notably, the POIs—BRD4, SMARCA4, and BTK—and the E3Ls—VHL, CRBN, and cIAP—featured diverse proteins of significant interest.
Refrences
by-NIKHIL MANE
Comments